Algorithms of Growth and Form
How do cells programed by tiny DNA molecules self organize to create the diverse shapes and patterns of tissue that make up life, what Darwin called “endless forms most beautiful and most wonderful?”. We believe that Nature has developed a recipe book of algorithms, mechanistic strategies for precisely programing tissue pattern and shape. These algorithms are implemented by systems at molecular, cellular, and mechanical levels with control architecture that makes them goal-directed and tunable.
Our lab use systems biology in zebrafish to determine the real control systems that orchestrate embryonic development. We use computational modeling to build models of how cellular automata can interact to build patterns and shapes. We use synthetic approaches to test our models in real life.
Our goal is to elucidate the set of design principles underlying growth and form. Such principles will form a scientific basis for tissue engineering, which is currently a black art, very unlike mechanical or aerospace engineering which are solidly grounded in physics, and very unlike how real tissues are made in vivo, and may one day lead to the creation of programable material.
The Megason lab has pioneered a suite of approaches for deciphering the design principles of how tissues are made including:
- in toto imaging– allows us to capture quantitative, single cell, dynamic, and anatomically intact data using the zebrafish embryo
- advanced computer vision algorithms and software for “digitizing” these data into cell-based virtual reconstructions
- mathematical modeling approaches for distilling the key principles of a process
- precision perturbations to test our models.
- we also want to test putative design principles using synthetic biology
Finding Design Principles
We have found a number of design principles for growth and form. In the neural tube, a textbook example of morphogen patterning, we found that morphogen gradients and interpretation are inherently noisy resulting in cell specification in mixed overlapping patterns (Xiong et al., 2014; Xiong et al. 2018). Noise in cell specification is corrected by sorting based on an affinity code of cadherins that gives different cell types different adhesive affinities (Tsai et al., 2020). Morphogen gradient formation is adjusted to account for differences in gene dosage and system size (we can make smaller embryos which have scaled down pattern) using an expansion-repression system in which Scube2 extends the gradient of Sonic hedgehog which represses Scube2 expression forming a negative feedback loop linking morphogen signaling and movement (Collins et al., 2018). Neural precursor proliferation and differentiation rates are balanced by putting differentiation rate under feedback control on cell packing density—as precursor density increases, cells get pushed away from the apical surface and in a Notch dependent manner are more likely to differentiate (Hiscock et al., 2018). The design principles in the neural tube are based on molecular, cellular, and physical control logic. Importantly they are used concurrently in a layered fashion allowing their strengths and weaknesses to be compensated. For example, morphogen patterning is noisy but globally ordered, while differential adhesion based self-organization gives only local order; together they can generate sharp domains of cell types (Tsai et al., 2019). Similar design principles are found in other tissues. In the early embryo, epithelial cell number and cell shape are linked by feedback between cell shape and cell division orientation (Xiong et al., 2015). Cell proliferation increase cell packing which makes cells more columnar and likely to divide perpendicular to the epithelium to relieve cell packing. As in the neural tube, this mechanism links cell proliferation and differentiation via cell packing but cell shape is molecularly transduced differently.
In the inner ear we found otic vesicle size is controlled by hydraulic feedback between fluid pressure and flux into the lumen (Mosaliganti et al., 2019) to achieve bilateral symmetry despite initial left-right variation (Green et al., 2017). Fluid pressure is relieved through pressure relief valves in which holes in the epithelium are made through partial removal of adherens junction and the holes covered with lamellae that burst open under pressure (Swinburne et al., 2018). The force that bends the epithelia to form the semicircular canals is generated by local extracellular fluid pressure that is shaped by filopodial tethers (Munjal et al., 2020).
The Digital Fish
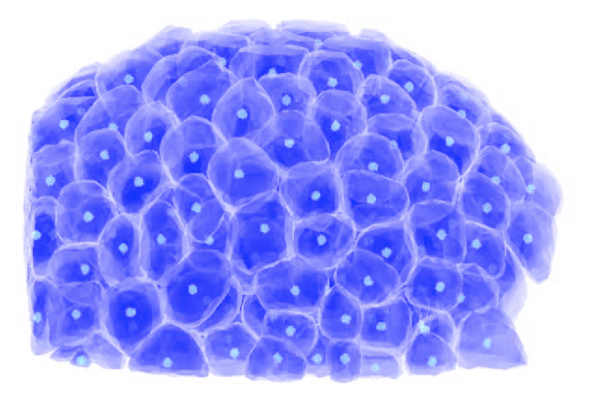
Our aspirational goal is the creation of a “Digital Fish”—a computational re-creation of the first day of zebrafish development in which the single-cell egg transforms to a fully patterned vertebrate body.
This“constructivist” approach to understanding the design principles of multicellular systems has three main parts:
- Upload real zebrafish development to create a Digital Fish that captures cell states across the first 24hrs of life using single cell sequencing and imaging
- Create a suite of models that mechanistically links cell states across time to create a Computable Fish by simulating gene regulatory networks, cell-cell signaling, and cellular mechanics
- Test and abstract the key principles at work to create a generalizable Recipe Book of Design Principles of Biological Form.
This constructivist approach is embodied in the original meaning of Richard Feynman’s quote “What I cannot create, I do not understand”? by which he meant that if we really understand a process, we should be able to re-derive that process. Our goal is to test the sufficiency of our understanding of the key processes of development by being able to recapitulate the the creation of zebrafish embryo in silico. We anticipate many failures along the way where our preconceptions about key mechanisms are insufficient, but these failures will point out gaps in our knowledge that we can then fill.